Rubber
The Pseudo-Elastic Response of Rubberlike Solids
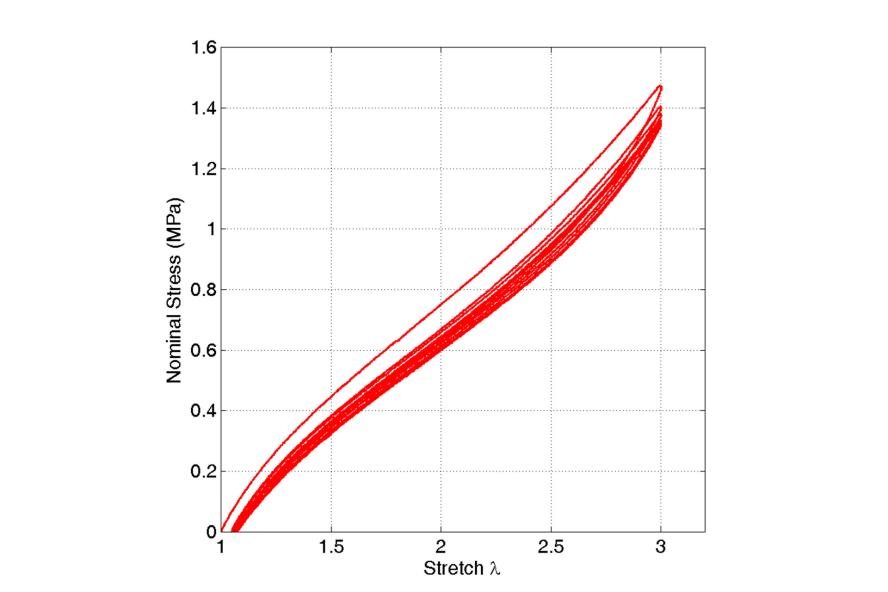
Figure 1: Pre-conditioning of a particle-reinforced dumbbell specimen with 1 phr of carbon black
with maximum stretch λ = 3.
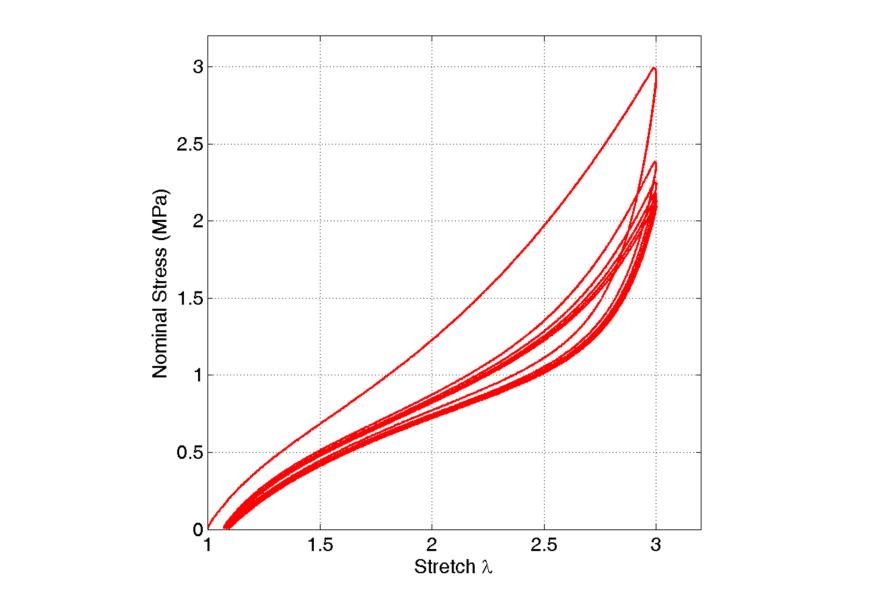
Figure 2: Pre-conditioning of a particle-reinforced dumbbell specimen with 20 of carbon black
with maximum stretch λ = 3.
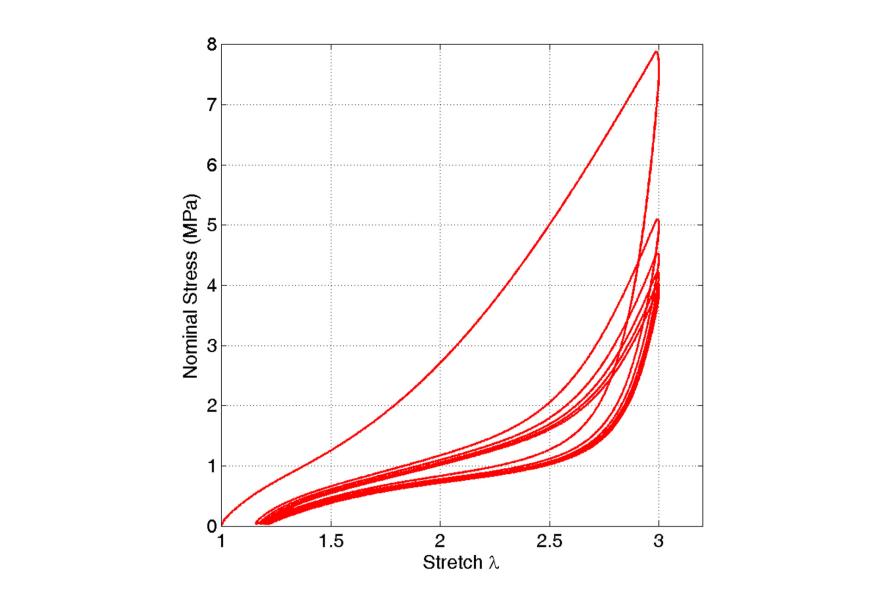
Figure 3: Pre-conditioning of a particle-reinforced dumbbell specimen with 60 phr of carbon black
with maximum stretch λ = 3.
Particle-reinforced rubbers exhibit a marked hysteretic response during unloading after loading in uniaxial tension, compression or shear, for example, i.e. the stress on unloading is significantly less than that on loading at the same strain.
The difference in the stresses corresponding to the same strain level under loading and retraction depends primarily on the proportion of filler in the rubber compound; for unfilled rubber the difference is negligible but it becomes very marked for elastomers with high carbon black content. The stress difference is greatest during the first loading-unloading cycle and approaches a fixed (strain-dependent) value after a number of cycles. This hysteretic behavior is not accounted for when the mechanical properties are represented in terms of a strain-energy function, i.e. if the material is modeled as hyperelastic.
This project is concerned with the formulation of constitutive models to capture certain inelastic effects in particle-reinforced rubbers subjected to loading-unloading cycles. As a basis for the modeling we use some recently obtained experimental data.
Publications
- Rubin MB, Dorfmann L (2020) A hyperelastic model for soft polymer foam including micromechanics of porosity. J Elasticity 135:205-220.
- Pancheri FQ, Dorfmann L Strain controlled biaxial stretch (2014) An experimental characterization of natural rubber. Rubber Chem Technol 87:120-138.
- Dorfmann A, Pancheri FQ (2012) A constitutive model for the Mullins effect with changes in material symmetry. Int J Nonlinear Mech 47:874-887.
- Dorfmann A, Ogden RW (2004) A Constitutive Model for the Mullins Effect with Permanent Set in Particle-Reinforced Rubber. Int J Solids Struct 41:1855-1878.
- Dorfmann A (2003) Stress Softening of Elastomers in Hydrostatic Tension. Acta Mech 165:117-137.
- Dorfmann A, Ogden RW (2003) A Pseudo-Elastic Model for Loading, Partial Unloading and Reloading of Particle-Reinforced Rubber. Int J Solids Struct 40:2699-2714.
- Drozdov AD, Dorfmann A (2003) A Micromechanical Model for the Response of Filled Elastomers at Finite Strains. Int J Plasticity 19:1037-1067.
- Drozdov AD, Dorfmann A (2001) Stress-strain Relations in Finite Viscoelastoplasticity of Rigid-Rod Networks: Applications to the Mullins Effect. Continuum Mech Therm 13:183-205.